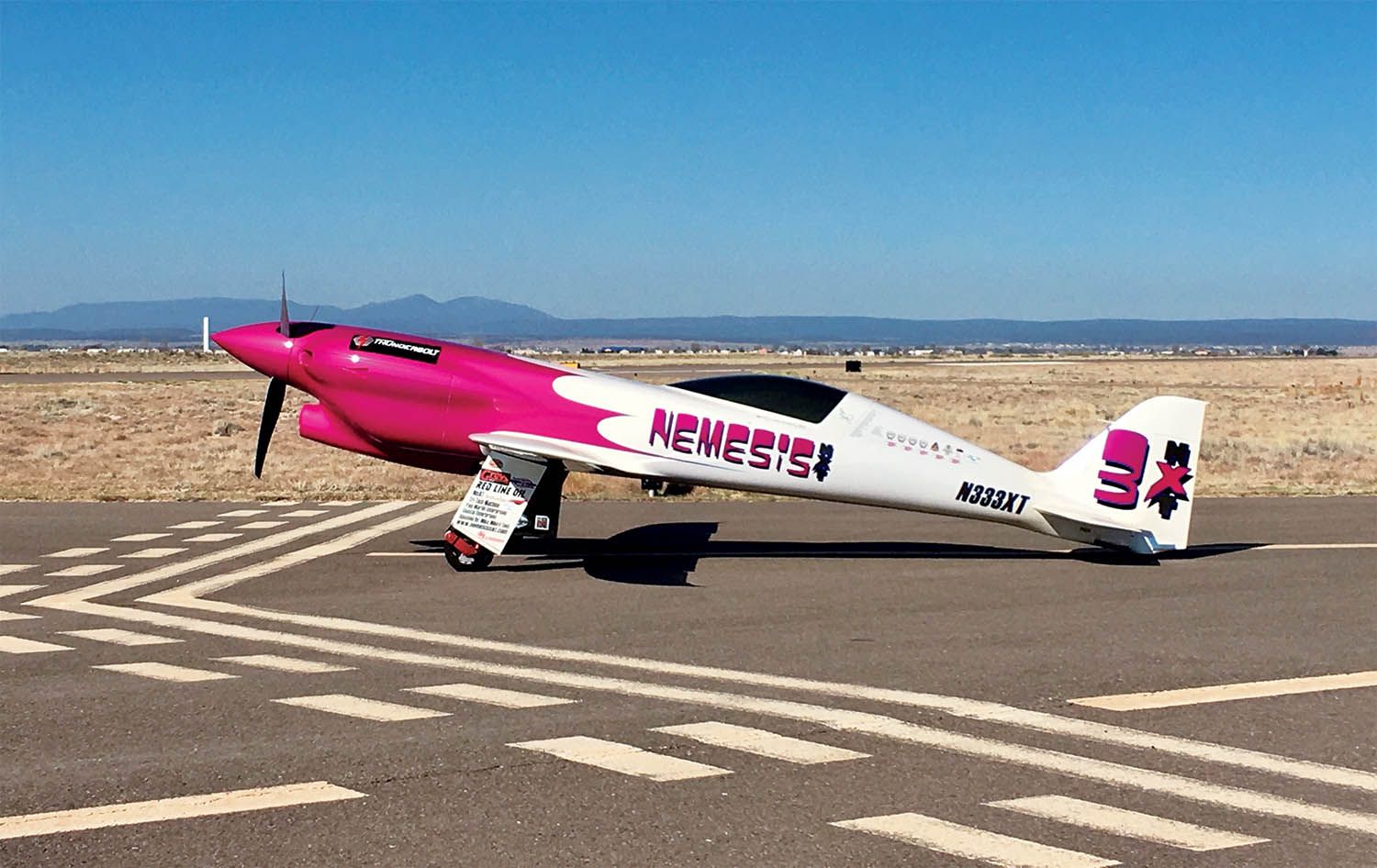
The engine of an airplane must produce enough power to meet the requirements of all phases of the mission.
First, the engine must accelerate the airplane up to liftoff speed during the takeoff roll.
Once the airplane is airborne, the engine must provide enough thrust to maintain flight and enough additional thrust beyond that to make the airplane climb.
In cruise or loiter, the engine must generate enough thrust to overcome the drag of the airplane and maintain level flight.
Ideally, the power needed to provide adequate performance for all three of these segments of the flight should dictate approximately the same size engine, but this is not always the case. The requirements for each phase of the flight are different, and which one drives the size of the engine will vary depending on the mission and specific configuration of the airplane.
Takeoff
During the takeoff roll, the engine must accelerate the mass of the airplane from rest to liftoff speed. The length of the takeoff roll is determined by the liftoff speed and the acceleration of the airplane.
Liftoff speed is determined by the stall speed of the airplane. The higher the liftoff speed, the longer the takeoff roll.
Acceleration during the takeoff roll is determined by the power-to-weight ratio. This ratio compares the maximum power of the engine to the weight of the airplane. The lower the power-to-weight ratio, the slower the acceleration, and the longer the takeoff roll.
While the aerodynamic drag of the airplane does reduce acceleration somewhat near the end of the takeoff roll, drag is less significant during the takeoff roll than during climb or cruise.
Relatively small changes in either liftoff speed or acceleration can have a surprisingly large effect on how long the takeoff roll is. This is because the distance required to accelerate to a given speed varies quadratically with both speed and acceleration.
For a given acceleration, the distance required to achieve a given speed is proportional to the target speed squared. For example, doubling liftoff speed would increase takeoff roll by a factor of four.
Similarly, the distance required to reach a given speed is inversely proportional to acceleration squared. Because of the squared relationship between distance and acceleration, cutting the acceleration in half will multiply the distance by a factor of four.
We see the effects of these phenomena when we compare ultralights or STOL aircraft to other types. The reason STOL aircraft use so little runway to take off is that they have low liftoff speeds and high power-to-weight ratios. They accelerate rapidly to a relatively low liftoff speed. With both squared functions working in their favor, they take off in a very short distance.
Climb
Once the airplane is off the ground and climbing the picture changes. A significant portion of the power developed by the engine now goes to generating thrust to offset the drag of the airplane and maintain airspeed. It is only the available power above that required to maintain level flight, otherwise known as excess power, that goes to driving the airplane uphill to make it climb.
The rate of climb of the airplane is determined by a quantity called “specific excess power” (Ps), which is the excess power above that required to maintain level flight divided by the weight of the airplane.
For the purpose of engine sizing, we can start out assuming constant weight and then look at the effect of changing power on rate of climb.
The excess power available is considerably less than the total power of the engine. For example, on a typical light trainer, the excess power at best rate of climb will typically be between one-fourth and one-third of the rated power of the engine.
This makes rate of climb much more sensitive to total engine power than other aspects of the airplane’s performance. Changing the rated power of the engine does not change the power required to maintain level flight, so any change in the power delivered by the engine goes directly into excess power. The percentage change in rate of climb will be much larger than the percentage change in rated power. For example, for the typical light trainer, increasing rated power by 25% will double the excess power available and therefore double the rate of climb (100% increase in climb).
Cruise
Power required to maintain airspeed is a direct function of the drag of the airplane. The higher the L/D ratio of the airplane, the lower the power required to cruise at a given speed.
To the first order, the power required in level flight is proportional to the cube of the airspeed. The first thing to realize from this is that simply adding power is not a very effective way to make an airplane go much faster. Doubling the power of an airplane will only make it go about 25% faster.
It is far more effective to use aerodynamic drag reduction to make an airplane go faster than it is to simply increase the power.
If we look at these two factors, we can get an idea of how cruise considerations will size the engine.
High-drag airplanes will never go very fast and may require quite a bit of power to achieve an acceptable cruise speed.
Airplanes designed to fly fast will require a relatively high power-to-weight ratio, even if they have low-drag airframes, because of the V-cubed relationship between speed and power.
Matching the Engine
The challenge for the designer is to take all of these considerations into account. Sometimes the three considerations—takeoff, climb and cruise—all dictate approximately the same size engine.
Light Sport Aircraft and trainers are a good example of this. They do not fly very fast and cruise speed is of secondary importance, so once the engine has enough power to give them acceptable takeoff and climb performance, it is sufficient for cruise. In other cases, however, one consideration dominates.
STOL airplanes are dominated by takeoff roll and initial rate of climb. They usually have relatively high drag configurations that will not fly very fast no matter what power is available. Accordingly, they tend to be overpowered for a reasonable cruise. They need a high power-to-weight ratio for takeoff acceleration and lots of excess power for initial climb in spite of their high drag, but they will cruise at a relatively low throttle setting for fuel economy.

Fast airplanes will often have engines sized by their top-speed requirements. Even with an aerodynamically clean, efficient airframe, high speed requires high power.
This can prove advantageous for takeoff and climb. High-speed airplanes tend to have high wing loadings and high stall speeds that would tend to increase takeoff roll. The extra horsepower needed to achieve the high cruise speed the airplane is designed for will also improve takeoff acceleration and help keep the takeoff roll down to an acceptable distance.
There is likely to be a large mismatch in power required for the three flight conditions in the case of an aerodynamically efficient but relatively slow airplane like a motorglider. Because of their low drag, these airplanes can successfully maintain level flight with very low power for their weight. An extreme example of this is the English Electric Wren, which is able to carry a pilot using an engine rated at 3.5 hp.
If the engine is sized to match this low-power cruise condition, the airplane is likely to be quite deficient in power for the takeoff roll, where the aerodynamic efficiency of the airplane does not matter and acceleration is strictly determined by the power-to-weight ratio.
Likewise in climb, where excess power over weight determines performance, an engine sized for cruise will not produce much excess power. Even at full throttle, the rate of climb is likely to be sluggish.
The trade here will really depend on the mission of the airplane. A self-launching sailplane will have an engine sized for takeoff and climb. The primary purpose of the engine is to replace the tow plane, not to cruise efficiently. The airplane is going to spend most of its time with the engine shut off and stowed or the prop feathered.
If an airplane is intended to cruise slowly or loiter efficiently under power, then the engine will be sized small to produce minimally acceptable takeoff and climb performance.
Thank you! Very well written. Great to re-ground myself on the basics.
Barnaby, keep these excellent informative bulletins coming. Keep on posting and if you can, keep on reposting them for their repeat aviation educational value from time to time. Both Northrop and our National Security are both proud and privileged to have you in our corner.