Probably no other aspect of all that noise on the far side of the firewall takes up as much of our time as engine cooling. Seemingly everyone has chased hot oil or cylinder head temperatures at some point, and among the more theoretical, the air- vs. water-cooled debate is a never-ending source of verbal sparing.
Perhaps a good place to start is why engines must be cooled in the first place. After all, the whole idea is to make heat and expand air in the combustion chamber, so wouldn’t it be best for the engine to run as hot as possible? The short answer is yes—witness jet engines—but we don’t have practical materials to build such piston engines out of.

No matter how it’s done, engine heat always ends up in the atmosphere, so every piston-powered aircraft will have cooling air inlets. Theory says a minimal number of air inlets branching to a multitude of consumers offers less drag, while experience shows multiple inlets dedicated to a single task are easier to design and control.
The Great Debate
As just noted, the big question is whether to cool with air or a liquid, namely water. Like all great debates, each side has excellent reasons for their prejudice, but we’ve come to view the question on whether one is engine- or airframe-centric.
Air cooling is easier for the airframe thinker. Air is free, it’s what we fly through. “Water cooling an airplane engine makes as much sense as air cooling a submarine,” they say. And the core of such thinking is valid; engine heat is rejected straight to the atmosphere. That’s where the heat is headed no matter if you water cool or air cool, so shedding directly to the atmosphere offers the lightest, simplest rejection path. Those designing airframes are pleased to ignore the complexities and weight of radiators, water, plumbing, and providing the access to service leaks.
On the other hand, engine people admit water cooling is more complex, but makes for a more efficient engine. They point to a cornucopia of engineering details facilitating higher cylinder pressures and more even temperatures, and say this is the way.
All said, today’s typical general aviation engines by both engineering truth, but increasingly by accident of historical precedent, occupy a niche currently well served by air cooling. But in our opinion, water cooling would be the best way forward should there ever be an economically viable future in advancing general aviation engines. That makes both methods worth our study.

Using aluminum sheet and flexible seals, traditional air cooling dams air between the cowling and cylinders in the high-pressure upper deck. Sealing the upper deck as tightly as possible to the cowling and baffles is the main construction and maintenance challenge with this system. Its advantage is easier construction and maintenance accessibility to anything inside the upper deck—namely spark plugs.
Air Cooling
Simplicity is the core attribute of air cooling. Heat is radiated directly to the atmosphere by increasing the surface area of the engine via cooling fins on the cylinders and cylinder heads, the remainder being oil cooled.
It’s not enough to leave the engine hanging out in the breeze, however. Air must be controlled as it passes around the cylinders to ensure the correct air mass, speed, and proximity to the cooling fins. As cooling air enters the cowling on a typical horizontally opposed engine, it is fenced in around the top of the engine by baffles tipped with flexible baffle seal material that mates the baffles to the cowling. Thus pooled in what is sometimes called the upper deck, the air slows and increases pressure.

Less leaky and thus more efficient, but more difficult to make and work around, plenum cooling encapsulates the upper deck independently of the cowling. Here air racing expert Andy Chiavetta mocks up the annular cooling inlet to his plenum-cooled UL Power LT-1 project. Chiavetta notes the plenum-with-annular- inlet combination gives design flexibility in determining cowling length and shape.
The only way out of the upper deck is via the cylinder fins. Various cuffs and inter-cylinder baffles force the air to wrap around the cylinders as much as practical, then allow the air to exit to the lower deck, which is simply the area under the engine. The lower deck is vented to the atmosphere by the cowling exit, which may have a hinged cowl flap to vary the size of the exit and thus the volume of air passing through the system.
Clearly it is imperative to have high air pressure in the upper deck and lower pressure in the lower deck or airflow will be compromised or even flow backwards out the cowling inlet.

Cooling airflow is almost always controlled at the system’s outlet, either by sizing of the exit or with a cowl flap. Exit flaps are workable with air- or water-cooling; this Subaru-powered RV has a generously sized and wide-opening cowl flap to control airflow through the radiator.
A crafty method of increasing cooling air velocity, and thus lowering the pressure in the lower deck, is exhaust augmentation. Cooling air exits the cowling via a channel—”boom tube” among friends—into which the exhaust system empties. The high-energy, fast-moving exhaust adds velocity to the exit air and replaces cowl flaps in the process. Beechcraft, Grumman, and Formula One racers have used augmenters to good effect.
Aside from cowl flaps and rubbing of the baffle seals against the cowling, there are no moving parts in air cooling systems so maintenance is pretty much confined to replacing chaffed or cracked cylinder baffles and seals. And such is the air-cooling advantage; it takes readily available air, guides it past the engine with a few pieces of sheet metal, and asks nearly nothing in weight or maintenance.

Specialized applications end up with specialized systems—look at the tiny cooling intake slits on this Pitts Special streamlined for pylon racing. The tradeoff with specialization is a narrowed operating range; hard summertime acro is likely not an option in this Pitts thanks to poor low-speed cooling.
The primary disadvantage of air cooling is it limits the engine’s specific output. Eventually you run out of surface area to reject heat through, thus limiting how much fuel the engine can burn. Limiting fuel limits power, and you end up with large-displacement, fuel-inefficient engines to make sufficient power.
There are exceptions, naturally. The Pratt & Whitney R-2800 and R-4360 radials employed forged, not cast, cylinder heads with thinly machined, not cast, cooling fins. This is an expensive way to make cylinder heads, but was one way of getting enough surface area to cool an engine making 0.89 hp/cu. in. of displacement. Another example was the Wright 3350, an even more extreme engine with many teething and operational issues. Capable of 1.04 hp/cu. in., the 3350 cylinder has very thin corrugated sheet aluminum stuffed between the usual cast cooling fins to increase surface area. By contrast, a 180-hp O-360 Lycoming makes about 0.50 hp/cu. in. and doesn’t come close in sophistication or ability to shed waste heat as the old radials.

Air-cooled engines are almost always downdraft cooled, but not always. Racer Tom Aberle favors updraft air cooling because it puts colder air on the hotter, exhaust valve side of the cylinder head. Here ace fabricator Andy Paterson builds the custom carbon fiber updraft cylinder baffles on biplane racer Phantom. They exit the cooling air through vents in the top of the cowling.
Workarounds for improving air cooling are spraying water over the engine for a powerful evaporative effect. One could say this is a form of water cooling, but in any event, the method is superb in sprint applications (racing, aerobatics, specialized climb scenarios), but requires too much water for the typical endurance work.
And it’s not just maximum power that’s affected by an air-cooled engine’s limited thermal efficiency; fuel consumption and range are compromised as well.
Shedding heat as they do directly to the atmosphere, air-cooled engines are more at the mercy of atmospheric variables. Large extremes in the sky’s temperature and density mean an air-cooled engine must withstand great variations in the rate of cooling. Yes, cowl flaps go far in controlling the rate of air cooling, but they can only do so much. The result is air-cooled engines expand and contract like balloons, so internal engine tolerances are goose-loose and operating limitations—avoiding rapid low-power descents for example—are tighter.
And while it’s not a law of physics, it does seem we have some to learn in general aviation about efficiently designing, sealing, and maintaining air-cooled engine cooling systems. If a water-cooled engine leaks coolant, it gets fixed; if an air-cooled engine leaks air through its upper deck, few people notice and fewer yet do anything about it. The result is designers opt for larger capacity, draggier air-cooling systems than we might need.

In the modern era the air- vs. water-cooling debate hinges on cost/simplicity as much as anything else. The relatively new UL Power engines combine the user friendliness of EFI fuel delivery with the simplicity of air cooling and direct drive to keep costs affordable, while the in-development EPS flat-8 champions power and efficiency and is water cooled.
Water Cooling
Liquid-cooled engines jacket the cylinders and cylinder heads with water. Heat is absorbed by the water, which is pumped to a radiator where the heat is shed to the atmosphere. An engine-driven pump circulates the water, and a thermostat sets the coolant’s minimum temperature.
Water is an excellent heat transfer agent and is the working fluid in almost all liquid cooling systems, although there are non-water fluids as seen in some Rotax engines. Where water is used, ethylene glycol is added as a corrosion inhibitor. Raising the pressure of the system via a spring-loaded vent increases the water’s boiling point and thus the system’s efficiency (the hotter the coolant and the colder the air passing through the radiator, the more heat is transferred per unit of time). Note, however, that if the coolant is run at very high temperatures, eventually the oil becomes the main coolant because it’s colder upon entering the engine and thus will absorb more heat.

Like some Porsche auto engines, Rotax has found water cooling the cylinder heads and air-cooling the cylinders is the best compromise between the most efficient engine and lightest, simplest installation. Air cooling fins on the cylinders are visible on this turbocharged 915iS; the “radiator” at left is actually the intercooler for the charge air. The smaller water radiator is out of sight here.
Liquid cooling’s major advantage is water is far denser than air. A smaller volume of water can transfer more heat than the same volume of air, so more cooling power can be packaged tightly around the engine. Also, dense water takes longer to warm up and cool down, thereby absorbing temperature spikes and localized hot spots much better than air. The resulting thermal stability yields a virtuous circle of improvements in engine design that result in higher specific output.

Aside from Rotax engines, auto conversions are another source of water cooling in modern Experimentals. The flat Subaru layout fits common cowlings, but one wonders about the airflow through the two front-mounted radiators due to the crowded area behind the heat exchangers.
Greatest of these are tighter piston-to-cylinder and piston ring gap clearances. The better sealed cylinder holds more cylinder pressure for greater power and efficiency, lets less oil past the rings to reduce detonation risk, dirties the oil more slowly, and means less oil needs to be carried aloft. Tighter pistons literally run quieter, enabling more ambitious knock sensor strategies in computer-controlled engines, too.

Oil coolers are commonly mounted directly to the rear cooling baffles because it is a compact, relatively easy fabrication job. This works well on low-performance aircraft such as this RV-9, but as speeds and heat rejection increase, mounting the cooler at an angle to organize airflow from the cooler toward the cowling exit help.
Another advantage is the ability to package greater cooling power around hot spots such as completely around the exhaust valve seat. Sophisticated internal water flow paths now possible from computer modeling also mean more even temperatures across the cylinder head. Engine temperature growth is thus vastly better controlled with liquid cooling; more precise tolerances throughout the engine are therefore possible. Again, it all adds up to more power and efficiency.
Liquid cooled engines can also be more physically compact and, even more importantly, rigid. That’s because the cylinders and cylinder heads can be cast in monolith banks rather than cantilevering out from the crankcase like a bunch of quivering porcupine spines. Because no cooling fins are needed, the cylinders can be more closely packaged, shortening the crankshaft—more rigidity—and the engine overall.
Need we also point out liquid cooling allows narrower form factors? A quick comparison between a V-12 and a radial will set that picture. That said, the now overwhelming realities of the horizontally opposed layout in general aviation means we won’t be seeing many razor-thin new inlines anytime soon.
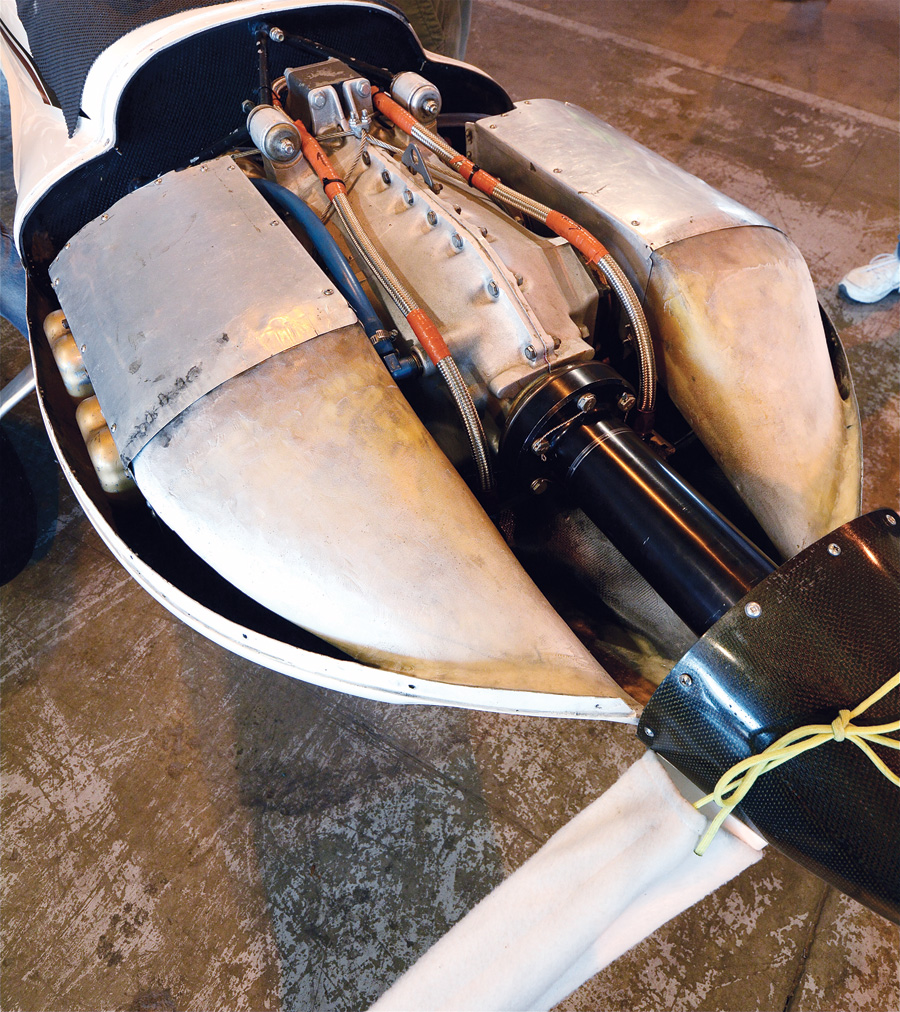
Streamlining counts, both inside and outside the cowling. Formula One racers employ long propeller extensions for a sleeker airframe, along with smoothly enclosed plenum cooling followed by exhaust-augmented air-cooling exit tunnels.
At the heat rejection end, to the airframe designer, a radiator is portable around the airframe and air can be efficiently ducted to it. Tricks such as the Meredith effect can reduce cooling drag, as can packaging the cooling exits into low pressure areas. Doing the same with air cooling is more difficult in practice.
Downsides to water cooling are its increased weight, complexity, and initial cost. There’s no getting around the increased weight, although greater fuel efficiency might recoup some of that in larger aircraft. Ditto complexity as a radiator, piping, connections, a pump and thermostat are all “extras” versus air cooling. But we must say the sometimes half-baked air-to-water cooled conversions of traditional Continentals and Lycomings result in needless complexity with silly cylinder-to-cylinder hose connections, externally-mounted thermostats, and other engineering affronts not found in a purpose-built liquid-cooled engine.

Eliminating sharp edges at the lower firewall air exit is another area racers such as Chiavetta have found useful in decreasing turbulence and organizing cooling airflow. Smaller, lower-drag cooling inlets and exits are thus possible.
Which brings us to a takeaway idea regarding water cooling: it takes a thoughtful integration of a purpose-built water-cooled engine into an airframe designed around it to realize all of liquid cooling’s advantages. And in our general aviation reality simpler, slightly less efficient air-cooled engines are often the lighter, less expensive, easier to live with choice.