
Editor’s note: Here is the rare opportunity to watch the engineer in his natural habitat. We met RV-9 builder Kurt Goodfellow the same day as Van’s Aircraft Chief Engineer Ken Krueger was undertaking test flights of the Wilksch-powered RV-9 and the factory’s own Lycoming-powered RV-9A. We were hoping to piggyback onto some of Krueger’s results, but when he offered to write a full evaluation for us—something we’d expected he’d keep inside the walls at Van’s—we said, “Heck, yeah.”
As the chief engineer for Van’s Aircraft, I have been following the development and maturation of the Wilksch Airmotive (WAM) diesel engines with more than passing interest. The size, weight and horsepower range of the WAM engine made it a possible candidate for installation into one of my favorite aircraft, the RV-9. While visiting the U.K. in 2006, I had a chance to see a Thorp T211 with a WAM-120 engine installed, but until October of 2009 I had never flown behind one. When Kurt Goodfellow, a builder from Boulder City, Nevada, called offering to let me look at and fly in his WAM-120 powered RV-9, I was eager to seize the opportunity.
For those not familiar with the WAM engine, it is a direct-drive, two-stroke, liquid-cooled, forced-induction diesel engine. Three inline cylinders reside beneath the high-mounted crankshaft; the propeller thrust line is close to where it is with the Lycoming despite the lack of a gearbox. (For a more complete description of the engine and the thought process that spawned it, see “The Man Behind the WAM Discusses Aero-Diesels” below.)
Side by Side by Side
It didn’t take a lot of time before the idea came of using the Van’s Aircraft RV-9A demonstrator to conduct a series of comparison flights with Goodfellow’s RV-9. By flying the two aircraft side by side, using GPS to measure speed and carefully accounting for the fuel consumed, we could eliminate a lot of variables and get a fairly good idea of the performance difference between the two with only a few flights. Because the aircraft were flying “same way, same day,” any atmospheric instability that affected one aircraft would equally affect the other. After digesting the data recorded during the comparison flights, it is possible to infer the “real-world” power output with the propeller efficiency and cooling drag accounted for.

Goodfellow earns his flying money by operating a rock-crusher business, and this alone makes him no stranger to diesel-powered equipment. But diesel power has been a hobby all of his adult life, and it includes several diesel conversions and a diesel-powered Bonne-ville streamliner. By his own admission, Goodfellow really likes diesel engines; in fact, it could be said that he is mad about diesels.
Knowing this, it is not surprising that his RV-9 has a WAM-120 powerplant. Mating the WAM-120 engine to an airframe designed for a Lycoming is quite a challenging task, and judging from the results, Goodfellow has met the challenge. The engine-mount design had already been worked out by Wilksch, and there are some WAM-120 RV-9As flying in the U.K., but Goodfellow felt some improvements could be made. Prior WAM installations had placed the two heat exchangers (radiators) stacked in series and placed low in front of the engine. This results in a short and almost certainly inefficient inlet duct. Goodfellow’s installation places the coolant radiator at the rear of the engine and low in the cowl, and the air-to-air intercooler is also placed at the rear of the engine but high in the cowl. Two cowl flaps are used. One is at the top with a forward-facing opening; it captures more inlet air, directing it through the intercooler. The second cowl flap on the bottom allows exiting air to be drawn through the coolant radiator. A single electric actuator powers the linkages that move the two cowl flaps simultaneously. He elected to install an MT three-blade, hydraulically actuated constant-speed propeller, which is typical of other WAM installations.
The comparison flights originated at Bishop, California. This location proved to be nearly ideal, as it was geographically convenient for both Goodfellow and me, the weather is consistently good, the airspace is uncongested—and there is a really good Thai restaurant in the terminal building. Additionally, the airport elevation is 4120 feet, and conducting the comparison flights at high altitude allowed the forced induction WAM engine to really shine.
The Test Plan
Preflight preparation involved fueling both aircraft, after which we could weigh each fully fueled as well as each pilot. The aircraft with the lighter pilot would carry ballast to make up for the difference in pilot weights.
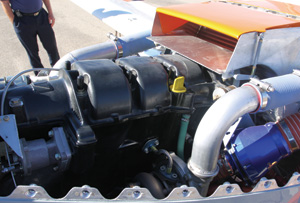
Because the aircraft in this comparison use different density fuels, it is also necessary to account for the difference in fuel weight and add ballast to the aircraft carrying the lower-density fuel. This approach is preferred to partially fueling one aircraft, because it eliminates variation due to estimation when partially filling a fuel tank. 100LL Avgas (at 6.02 pounds/gallon) is less dense than Jet A (at 6.76 pounds/gallon). Given that the RV-9 fuel-tank volume is 36 gallons, the Lycoming-powered RV-9A had to carry 27 pounds of ballast to compensate for the denser fuel carried by Goodfellow’s RV-9. The total ballast carried by the Lycoming RV-9A was 58 pounds: 31 for the pilot and 27 for the fuel.
The test takeoff weight for the Lycoming-powered RV-9A was 1522 pounds (1083 pounds empty plus 217 pounds avgas plus 164 pounds pilot plus 58 pounds ballast). The test takeoff weight for the Wilksch-powered RV-9 was 1465 pounds (1027 pounds empty aircraft plus 243 pounds jet fuel plus 195 pounds pilot).
The Flights
Three specific test flights were conducted to look at particular aspects of the Wilksch-powered RV-9’s performance.
Flight No. 1: This was a side-by-side flight comparing takeoff distance, climb rate and maximum speed at three different altitudes. The two aircraft take off side by side, accelerate to a preselected IAS, and climb at that speed to a pre-selected altitude. The elapsed time from brake release to target altitude for each aircraft is recorded.

With both aircraft at altitude, the aircraft achieve stable side-by-side flight at preselected cruise speed. Once established in steady side-by-side flight, both aircraft go to full power and full rpm while holding heading and altitude. Allowing 2 minutes for speeds to stabilize, the GPS ground speed (GPS GS) and indicated airspeed (IAS) for each aircraft are recorded. This test was conducted at 8000, 10,000 and 12,000 feet MSL. After recording data, both aircraft return to base and refuel. Fuel required to fill each aircraft is recorded as well as total flight time.
Flight No. 2: Another side-by-side flight but made into a short cross-country of approximately 1 hour total time. In this test, the two aircraft fly the entire time side-by side with the slower aircraft setting the pace. For the sake of fairness, the aircraft with the non-standard engine is allowed to select the flight profile (climb speed, cruise altitude, cruise power setting). This gives the non-standard aircraft the maximum advantage, or stated another way, does not inadvertently put it at a disadvantage. Again, because they are in the same atmosphere at the same time and altitude, the variables are greatly reduced.
After the flight, both aircraft are fueled and the amount of fuel consumed by each aircraft is recorded along with the flight time. Because the flight was to a selected point and back, the distance flown is also recorded. For this comparison the two RV-9s flew from Bishop, California, 83 miles north to Hawthorne, Nevada, (overflight only, no landing) and then back to Bishop.
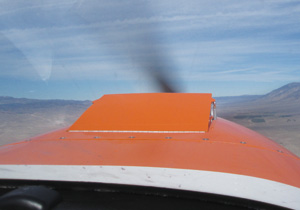
Flight No. 3: This was a subjective, “overall impressions” evaluation of the Wilksch-powered aircraft. In addition, we also performed two timed power-off descents so that the difference in drag between cowl flaps open and cowl flaps closed could be calculated. (By appearances, the Wilksch installation appears to have more cooling drag, particularly with the larger upper cowl flap open. This test would allow us to assess the degree of that penalty.)
Diesels are reputed to be heavy, but this was not the case here. The WAM-120 engine/MT prop RV-9 is 57 pounds lighter than the RV-9A with the Lycoming O-320/Hartzell two-blade, constant-speed prop. That’s a big difference in empty weight, but it’s not surprising. The WAM-120 engine is best compared to the Lycoming O-235 both in terms of power output and weight.
Performance Comparison, Flight No. 1
Rate of climb: Taking off from 4120 feet MSL, accelerating to 90 mph IAS, and climbing to 12,000 feet. For this test, the WAM RV-9 required 9 minutes 9 seconds versus 7 minutes 30 seconds for the Lycoming RV-9A. Average climb rate was 861 fpm for the WAM RV-9 versus 1051 fpm for the Lycoming RV-9A.
Cruise speed: At 12,000 feet the WAM-120 RV-9 was 16 knots (true) slower than the Lycoming RV-9A. At 10,000 feet, that difference was 13 knots, and at 8000 feet, the Wilksch was 19 knots slower. This difference in speed is not surprising, as not only does the Lycoming RV-9A have more horsepower, it also has a more efficient two-blade propeller. Because the WAM engine has forced induction and can make its continuous rated power at practically any altitude, it is expected that the speed disadvantage would diminish with increasing altitude, and that’s pretty much what we saw. The surprise was that there was a greater difference in speed at 12,000 feet than at 10,000 feet, attributable to experimental error. Welcome to the real world!
Fuel Consumption
Because most RVs have engines that make shaft power by burning gasoline, the comparison of fuel consumption generally ends at the number of gallons. In this instance where we have an RV with an engine that burns jet fuel, we consider two additional ways of comparing fuel consumption: fuel energy content and fuel cost. For this comparison, the energy content of 1 gallon of 100LL is assumed to be 120,000 BTU compared to the 135,000 BTU assumed to be in 1 gallon of Jet A. At the Bishop Airport on the day of the comparison flights, 100LL cost $4.387 per gallon and Jet A cost $3.752 per gallon. Comparing the number of BTUs consumed is the best way of measuring efficiency, but the comparison that hits closest to home is fuel cost. (See Table 1 for details.)
Cowl Flap Drag
For this test the total weight of the WAM aircraft, fuel, pilot and passenger was 1615 pounds. Power-off glide at 100 mph IAS with cowl flaps closed resulted in an average descent rate of 817.5 fpm. Given the gross weight of 1615 pounds, this tells us that the aircraft requires approximately 40 thrust horsepower to maintain level flight at 100 mph IAS.
Power-off glide at 100 mph IAS with cowl flaps open resulted in an average descent rate of 990 fpm. Assuming the same gross weight as during the cowl flap closed glide, approximately 48.5 thrust horsepower is required to maintain level flight at 100 mph IAS with cowl flaps open. This means that at a typical climb speed the open cowl flaps are costing 8.5 thrust horsepower or, assuming an 80% efficient propeller, 10.6 shaft horsepower.
Overall Impressions
The WAM-120 engine is a very good match to the RV-9 airframe, arguably better than the O-320 because it is very light but with adequate power. The aircraft can cruise fairly fast and because of forced induction, the fuel economy at altitude is excellent. The constant-speed prop yields good operational flexibility by allowing the engine to make its rated horsepower during takeoff and climb. The cabin noise and vibration levels are comparable to that of a Lycoming-powered aircraft.
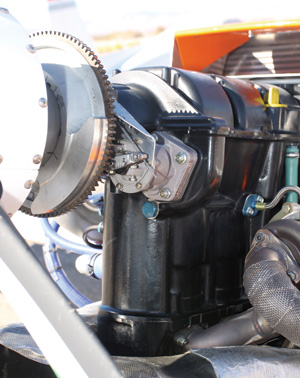
Operating the engine is simple, as there is no manual mixture control. However, this is offset slightly by having to open and close the cowl flaps. The additional drag of the open cowl flap is measurable and would explain why the climb rate of the WAM RV-9 was not as good as might otherwise be expected. (For perspective, consider that the climb rate of many “high-performance” certificated aircraft is comparable to that of the WAM RV-9. It’s just that the “big engine” Lycoming RV-9A with the constant-speed prop has climb performance most people only dream about.)
I describe the engine as being built on a solid foundation. As a direct-drive powerplant it eliminates all of the compromises associated with gearing, uses a hydraulic constant-speed prop to get the most output from the engine regardless of flight condition, and it has the simplicity of all-mechanical systems, thereby eliminating the need for electronics (or even electricity) to keep running optimally.
Goodfellow’s mating of the WAM engine to the RV-9 airframe builds on the engine’s solid foundation in that the installation includes reasonable size and length ducts for cooling air, has effective cowl flaps for regulating engine temperatures and is a handsome installation with the cowling on and off. This good foundation shows through in the aircraft’s good looks, efficiency and simplicity of operation. By starting with the well-proven RV-9 airframe and then installing the light and efficient WAM engine, Goodfellow has crafted an amazingly capable aircraft.
Flight No. 1 | |||||||
---|---|---|---|---|---|---|---|
Aircraft | Fuel Volume | Fuel Energy Content | Fuel Cost | Rate of Climb, Avg | Speed Delta, 8000 feet | Speed Delta, 10,000 feet | Speed Delta, 12,000 feet |
WAM RV-9 | 5.6 gallons | 751,950 BTU | $20.90 | 861 fpm | -19 mph TAS | -13 mph TAS | -16 mph TAS |
Lycoming RV-9A | 6.2 gallons | 744,000 BTU | $27.20 | 1061 fpm | |||
Flight No. 2 | |||||||
The cruise altitude for the 166-s.m. roundtrip from Bishop to Hawthorne was 9000 feet, and it took 1 hour 7 minutes from takeoff to touchdown, resulting in an average ground speed of 149 mph. | |||||||
Aircraft | Economy by Volume | Econ by Energy | Econ by Cost | ||||
WAM RV-9 | 33.2 statute mile/gallon | 4066 BTU/statute mile | $0.113/statute mile | ||||
Lycoming RV-9A | 23.9 statute mile/gallon | 5017 BTU/statute mile | $0.183/statute mile |
For more information, visit www.wilksch.com.