In the early days of aviation, designers believed that the total drag of an airplane could be calculated by simply measuring (or calculating) the drag of each component (wing, body, tail, landing gear, etc.) separately and then adding them together. They quickly learned that this method was not particularly accurate, and almost always underpredicted total drag. The reason for this inaccuracy is that the “summation” method of drag estimation ignores the effect of combining the isolated components into a complete airplane.
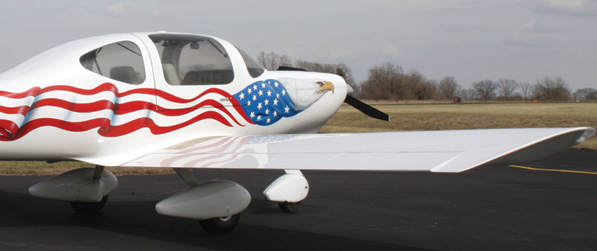
Calculating Drag Effects
The drag change caused by the aerodynamic interaction of the various components of the airplane is called interference drag, which can make up a substantial portion of the total drag. For preliminary design performance estimation, interference drag can be taken into account by calculating the drag of the airplane by summation of each component drag, and then adding between 6% and 10% to the parasite drag calculated by summation. The interference drag of a poorly designed airplane can be much higher than this, but it is rarely less than 5%, or even 6%, for a well-designed, clean airplane.
The airflow over an isolated component of an airplane is not the same as when that component is combined with others to form a complete airplane. Each part of the airplane affects the air flowing over it, which in turn influences the airflow over the other parts of the airplane. The interaction is especially large in the neighborhood of junctions between components. This interaction and alteration of the airflow affects the drag of the airplane. In most cases it will increase drag, sometimes dramatically.
In a few cases, the interaction of components may be favorable and actually decrease drag. On certain military airplanes, drag is actually lower with wingtip-mounted missiles or fuel tanks in place rather than after they have been jettisoned. The wingtip-mounted components act like endplates and increase the span efficiency of the wing. This produces a reduction in induced drag that is large enough to offset the increase in parasite drag caused by the tip tank. Winglets are another example of favorable interference. A properly designed wing-winglet combination has less drag than the wing alone, due to the winglets’ effect on induced drag.
Despite these exceptional cases, the effect of flow interference generally increases drag. An example of how powerful this effect can be is the drag of a simple “T” formed by two intersecting streamlined struts similar to the intersection between the mail lift strut and jury strut on a high-wing airplane. The drag caused by interference is equivalent to the drag of a section of isolated strut that is 10 times the strut thickness in length. This is caused by flow separation induced in the corners, at the joint between the struts. The addition of a fairing can reduce the interference drag to only a few percent of the total drag of the two struts.
This example illustrates two important facts about aerodynamic interference: First, interference effects can be quite large. Second, they can be minimized or eliminated by proper design.
Wingroot Junctions
The junction between the root of the wing and the side of the fuselage is one of the primary problem areas for interference drag. A poorly designed junction can cause a large increase in drag, particularly in climb and economy cruise where the lift coefficient is high.
In general, junctions on the upper surface of the wing are more critical than lower-surface junctions. The airflow over the upper surface of the wing is much more sensitive to interference, which might cause premature separation or stall.
Better wing-to-fuselage junctions are an argument in favor of high-wing airplanes. In a properly designed high-wing configuration the fuselage side does not cut the upper surface of the wing, and so it does not interfere with airflow over it. The wing/fuselage intersection on most high-wing airplanes affects only the less-critical lower surface of the wing. While this is advantageous, experimentation has shown that, with proper design of the fuselage and the wing-to-fuselage junction fairing, low-wing airplanes can have interference drag that is no higher than that of high-wing airplanes.
Because the airflow over the upper surface of an airplane wing is so sensitive, careful design of the wing-to-fuselage junction area is vital in keeping interference drag to a minimum. One recurring myth in the popular aviation press is that if the angle between the wing surface and the fuselage side (in the front view) is 90 or more, the junction does not require a fillet or fairing. This is most definitely not true. There are many airplanes with 90 or near-90 wing junctions that could benefit significantly from the addition of a root fairing. A basic rule of thumb is that if the junction occurs at an angle of less than 90, then a fairing is usually necessary. However, this does not mean that a fairing is unnecessary when the junction angle is greater than 90.
The plan (overhead) view shape of the fuselage in the area of the wingroot can also have a large effect on the flow over the wingroot. On many light airplanes the fuselage sides begin to pull inward rapidly immediately aft of the cabin area. Unfortunately, this occurs ahead of the trailing edge of the wing in an area where the surface of the wing is sloping downward and pulling away from the direction of the airflow. The air may not be able to follow the slopes of both the wing and fuselage at the same time, which will cause the flow to separate, with the result being increased drag and premature stall.
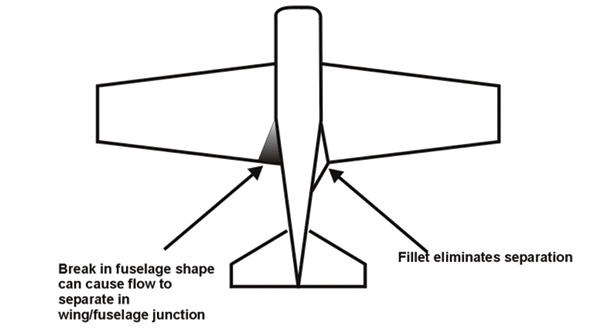
Most wings stall from the root first, so a poor root junction design can cause some portion of the wingroot to be stalled even at climb angle of attack. This premature root stall will hurt both the rate of climb and stall speed of the airplane. Figure 1 shows that the separated zone can be eliminated by the use of a proper fillet, even when the fuselage is pulling in over the wing. Another solution to the junction problem is shown in Figure 2. If the fuselage has its widest point at or near the trailing edge of the wing, then premature flow separation at the wingroot is much less likely. The Thorp T-18 and the Questair Venture both use this type of fuselage shaping to minimize separation at the wingroot junction.
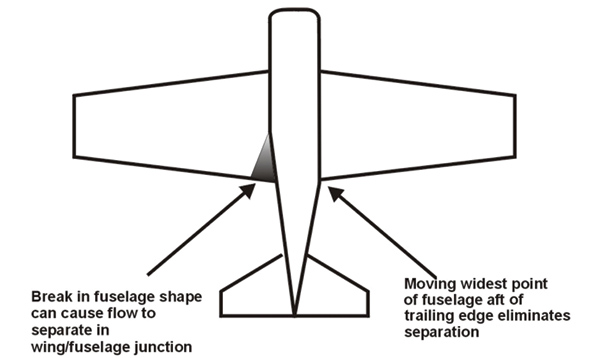
Fillets to the Rescue
Even in a situation where significant separation is not expected, some filleting is still a good idea. Air flowing in a corner between two surfaces is slowed by skin friction with both surfaces. This causes the boundary layer to thicken and generate more drag. It also may produce early separation. Radiusing the corner with a fillet will help in two ways: First, the wetted area near the corner is reduced. Second, the air will not have to rub against both surfaces forming the corner and will produce less skin friction drag.
Fillets are particularly effective in areas where surfaces meet at angles significantly less than 90. One common example of this situation is at the wingroot of a low-wing airplane with a rounded fuselage cross-section and/or dihedral in the wing. The addition of a fillet will clean up the flow in the corner area and reduce the drag of the airplane. Proper design of the fuselage can minimize the interference drag caused by the wingroot junction and reduce the size of the root fairing required. A high-wing configuration with some dihedral is an example of a low interference drag design. The upper surface of the wing is uninterrupted and the lower surface joins the fuselage at a large angle, thus minimizing the undesirable corner effects on the airflow.
There are a few general rules for the design of low interference drag wingroot junctions:
- Keep the included angle between the wing surfaces and the fuselage as large as possible. This is particularly important on the wing upper surface.
- n the top view, the sides of the fuselage should not pull in sharply at the area of the wing-to-fuselage junction. Without the fillet, the junction is likely to exhibit separation in the shaded areas at relatively low angles of attack. Airflow may be separated during climb and slow cruise. This early separation will hurt rate of climb and increase stall speed. If other design considerations force the fuselage to have this type of shape, then a large fillet will eliminate the root flow separation. If the fuselage has a constant or slightly increasing width in the area of the wing, the chances of early flow separation at the root are reduced. A fillet may still be required, but it can be smaller, and the penalty for not filleting this type of wingroot configuration will be much lower than it would be for a configuration in which the fuselage pulls in sharply over the wing.
- From the overhead view of the wing, the fuselage should have no corners or areas of large curvature. A corner or sudden change of direction in the shape of the fuselage can induce separation even without the wing attached to the fuselage. The addition of the wing makes airflow more sensitive and easy to separate, particularly in the region just above the wing. If the airflow does separate at the corner it will cause separation over the upper surface of the wing in addition to the fuselage side. If other considerations require a break in the fuselage, the break point should be aft of the wing to reduce the chances of separation and interference drag. Corners are not good from the drag point of view in any case, but if they are required, then they should be placed where they do the least harm.
- Avoid gaps between the wing and fuselage. Any gap between the root of the wing and the fuselage should be well sealed. Air leaking through such a gap, if it is left unsealed, can markedly increase the drag of the airplane. Leakage can produce flow separation on both the wing and fuselage where they join. It can also increase the induced drag of the airplane. The gap acts somewhat like an additional wingtip, which decreases the effective aspect ratio of the wing by reducing the span efficiency and increases the induced drag of the wing.
- Avoid situations where the wing is pushing air one way and the fuselage is pushing it the other way. An example of this is an aft-cambered wing mounted low on a fuselage that pulls up sharply just aft of the wing. The air leaving the wing is deflected downward. The air flowing over the bottom of the fuselage, just inboard of the wing is deflected upward by the fuselage. Clearly, the air right at the junction will be severely confused and will likely separate or form a vortex, causing additional drag. Proper design of the wing-to-fuselage junction is particularly important if the designer chooses to use one of the new generation aft-cambered airfoils. Because of their camber distributions, these aft-loaded sections are likely to be quite sensitive to the perturbation of airflow produced by the fuselage. A good wingroot junction is essential to prevent separation.
Wing-junction design seems to be one of the most neglected areas in light airplane design. Many production and homebuilt airplanes lack any form of root fairing at all and many others have root treatments that are inadequate to minimize interference drag. Your homebuilt’s wing-to-fuselage junctions are just as vital to performance as any multi-engine airplane’s tail-surface-to-fuselage and wing-to-nacelle junctions.