
Not every airplane is designed to perform intentional spins, but that doesn’t mean the designer can safely ignore the effect of the vertical tail and rudder on the spin characteristics of the airplane. Light airplanes certified under U.S. FAR Part 23 must meet the following rule even if they will be placarded against intentional spinning once certified.
Sec. 23.221— Spinning
(a) Normal category airplanes. A single-engine, normal category airplane must be able to recover from a one-turn spin or a three-second spin, whichever takes longer, in not more than one additional turn after initiation of the first control action for recovery, or demonstrate compliance with the optional spin resistant requirements of this section.
This one-turn spin is really still an incipient spin, and some airplanes certified under this rule cannot recover from a fully developed spin. Although I personally have reservations about this, the important point of this rule is that the pilot must be able to recover control of the airplane at the beginning of the typical stall/spin accident scenario, which usually starts at relatively low altitude and never progresses to a fully developed spin.
Aerobatic airplanes, and airplanes used to train spin recovery technique, must be able to be placed into a fully developed spin and recover. In my opinion, it is preferable for any airplane in which stalls are taught to be recoverable from a fully developed spin, even if they are prohibited from intentional spinning.
The characteristics of the vertical tail and rudder are critical to the flying qualities of the airplane at high angles of attack. Both the control power provided by the rudder and the yaw damping provided by the vertical tail are important.
Rudder for High Angle of Attack Control
As angle of attack increases from the first incipient stall through full stall, the ailerons become ineffective. As the stall progresses, roll damping either disappears or becomes unstable. At this point the airplane is likely to drop a wing and roll off into the beginning of a spin entry.
The ailerons are ineffective, and aileron roll control may actually be reversed due to the effect of aileron deflection on how the stall progresses. If the pilot tries to pick up the dropping wing with opposite aileron, adverse yaw will tend to yaw the airplane into the developing spin.
Because of these effects, the rudder is the primary surface for lateral control as the aircraft approaches the stall. Pilots are trained to keep the yaw rate to zero and “pick a wing up with rudder” if the airplane starts to roll.
The rudder is the most important control used to arrest an incipient spin and keep the airplane from departing from controlled flight.
In a developed spin, the proper control inputs for recovery vary depending on the configuration of the aircraft. For general aviation aircraft, particularly single-engine airplanes, the rudder is the primary control for spin recovery.
In 1936, NACA published Technical Note 555 that described the “basic spin-recovery procedure” that is still taught today. The classical spin recovery technique for single-piston-engined tractor airplanes from NACA TN 555 (1936) is:
- Briskly move the rudder to a position full against the spin.
- After the lapse of an appreciable time, say after at least one-half additional turn has been made, briskly move the elevator to approximately the full-down position.
- Hold these positions of the controls until recovery is effected.
Note that full rudder against the direction of rotation is the first and most important control input. Accordingly, the vertical tail should be designed to ensure that the airplane retains rudder control effectiveness even at post-stall angles of attack.
Yaw Damping
A spin is a combined rolling and yawing motion. The steady-state rotation rate is determined by the balance between the pro-spin force generated by the asymmetric stalling of the wings and the anti-spin yawing moment due to yaw damping.
The yaw rotation in the spin pushes the vertical tail sideways through the air. The sideways motion of the tail rotates the apparent wind and generates an angle of attack on the tail. This causes the tail to generate a side force that generates a yawing moment that opposes the yaw rate.
The greater the yaw damping the vertical fin generates, the slower and steeper the spin will be.
The slower the rotation rate in the spin, the less anti-spin yawing moment from the rudder will be needed to stop the rotation. A steeper (more nose-down attitude) spin is happening at a lower angle of attack, so less nose-down rotation will be needed to break the stall and eliminate the pro-spin forces from the wings.
If there is enough yaw damping, the airplane may self-recover if the pilot releases the pro-spin controls. (Note that this is not true for many types and is not a standard spin recovery procedure.)
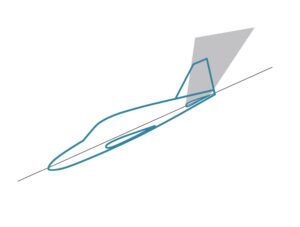
Airflow Over the Vertical Tail
It’s important to ensure a flow of clean high-energy air over the vertical fin even post-stall. This requirement is why some fighters have twin vertical fins, since moving the fins outboard gets them out of the separated wake of the nose and body at very high angles of attack.
For airplanes with the conventional single-fin tail configuration, the relationship between the fin, rudder and horizontal tail is very important.
In a developed spin, the horizontal tail and elevators are at least partially stalled and shed a low-energy separated wake above the tail. If too much of the fin and rudder are buried in low-energy separated flow, the vertical tail will become ineffective. The fin will not generate enough side force to produce yaw damping, causing the spin to be faster and flatter, and the rudder will not be able to generate enough yawing moment to stop the rotation of a spin.
A simple method of estimating how much of the fin and rudder is blanked by the tailplane wake is shown in Figure 1. On a side view of the airplane, draw a line from the leading edge of the tailplane upward at an angle of 60% above horizontal. From the trailing edge of the tailplane, draw a second line upward at an angle of 30%. The airflow in the area between these two lines is likely to be separated in a developed spin. Any fin or rudder area between the two lines will be blanked by the tailplane and will be ineffective.

Figure 2 shows how some combinations of tail position and fin planform affect how much of the vertical tail remains effective in a spin. Note in particular that having side area (fin and/or fuselage) and rudder below the horizontal tail gives the best spin recovery characteristics. If you look at aerobatic airplanes, almost all of them share the following characteristics:
- Fin and rudder have little or no sweep-back.
- The rudder is tapered and has its maximum chord down low to maximize the rudder area below the horizontal tail.
- The horizontal tail is mounted high on the fuselage to expose fuselage and rudder area below it.
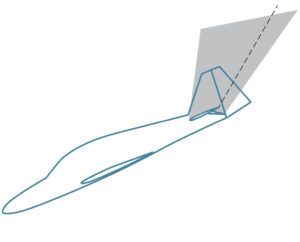
The position of the elevators affects the flow over the rudder at high angles of attack. Deflecting the elevators down can significantly increase the blanking of the rudder by the tail wake as seen in Figure 3. There is at least one airplane that is used for aerobatic training where this effect is so significant that the spin recovery procedure for that type is to keep the stick full aft until opposite rudder stops the spin rotation before moving the stick forward to reduce angle of attack. (Note that this is not a standard recovery technique and should not be used on any other type of airplane unless it is specifically required in the POH.)
For a detailed summary of NACA research on tail design and spin recovery see NACA-TN-1045: “Tail-Design Requirements for Satisfactory Spin Recovery” by Anshal I. Neihouse, Jacob H. Lichtenstein and Philip W. Pepoon (https://ntrs.nasa.gov/citations/19930081653).
Well, you lost me when you stated the rudder is the most important flight control for spin recovery – if the stall can be broken the spin will stop. …I might be skidding down (with the rudder still deflected into the spin) but the rotation will have stopped. If I could only pick one control surface to break the stall (and consequently the spin), its the elevator!
The most important control used to arrest an INCIPIENT spin and keep the airplane from departing from controlled flight is the elevator, not the rudder. Indeed, if you don’t do something to get the AOA down, then applying rudder merely selects the direction of rotation – and can reverse the direction of spin entry without restoring controlled flight.
Mike, T, I am afraid you are both mistaken. Rudder stops the spin, elevator push makes sure you are ready to recover when the spin stops. Otherwise we would have called it deep stall instead of spin