Sometimes, despite the best efforts of the designer, the aerodynamic characteristics of an airplane are not as good as desired. Often, problems are caused by flow separation. Recontouring the surface of the airplane to eliminate this separation is expensive, particularly if the problem appears after the airplane is completed and flying. At that stage, an add-on fix that can reattach the flow on the existing shape is most desirable because it offers the chance to fix the problem without a redesign of the airplane.
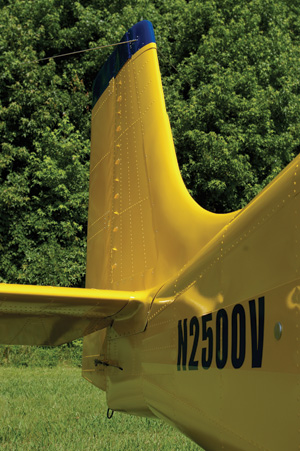
One such fix is a device called a vortex generator. There are several types of vortex generators, but the most common is the vane type. These are small, low-aspect-ratio blades that are mounted perpendicular to the skin of the airplane. The vortex generator acts like a low-aspect-ratio wing. It develops lift normal to the oncoming flow and sheds a vortex off of its free tip. The tip vortex of a VG stays close to the surface of the airplane as it moves aft.
The vortex moves air that is near the airplane skin up away from the skin while at the same time moving air from the outer flow down closer to the skin. When separation is imminent, the air in the boundary layer right near the skin is out of energy. It is moving very slowly, and will soon stop and reverse direction, causing separation. The air in the outer flow is moving fast. The vortex created by the vortex generator moves some of this high-energy air down into the tired boundary layer, and moves some of the tired air away from the surface. This increases the speed of the air in the boundary layer and delays separation of the flow.
To work properly, the VG must be able to affect the air outside the boundary layer. It must be placed slightly upstream of the point where the flow would separate without the VG. If the flow separates upstream of the VG, it will be submerged in the separated flow, and will be unable to mix clean outer-flow air into the boundary layer.
Uses of Vortex Generators
Vortex generators, when installed in groups, can help eliminate separation and solve a variety of aerodynamic problems. Recently, VG kits have appeared to tame the low-speed behavior of some production twins including the Beech Baron and some of the more popular Cessna twins. Vortex generators provided a cure for the trim-change problems encountered by several common canard and tandem-wing homebuilts in rain, and were also used on the canard of the world-circling Voyager. While VGs are getting a lot of attention recently, they have actually been around for a long time, and are a feature of many well-known commercial and military airplanes.
Shock Stall
The first VGs that I am aware of were used to delay flow separation behind shock waves on the wings of early high-speed airplanes. This phenomenon, which is sometimes called shock stall, was a major problem for the designers of early high-speed jets. Vortex generators were tested by NACA on the Douglas D-558-1 Skystreak research airplane, and were found to significantly improve the lateral stability of the airplane at high subsonic speeds.
The first production airplane with VGs was the Boeing B-47. At the time, the B-47 was a truly radical design, being the first large swept-wing airplane to fly. Although it far outperformed other contemporary bombers, the B-47 had a tendency to pitch up when maneuvered at its cruise Mach number. This pitch-up was caused by the formation of a shock wave on the outboard portion of the wing. The shock induced separation of the flow on the outer part of the wing, causing it to lose lift and pitch the nose of the airplane up. The addition of VGs in the area of the shock eliminated the separation, and cured the pitch-up. Boeing still makes use of VGs as a way of tailoring the aerodynamics of its wings, and most Boeing airliners have at least a few of them on the upper surface of the wing.
After-body Separation
On military aircraft, some separated flow on the after-body of a fuselage or pod is common. Flow separation on after-bodies occurs when the after-body is too bluff, and pulls in too sharply for the flow to follow. This is particularly common if the airplane has either an aft cargo door for air-dropping cargo, or if there is a radome or other sensor antenna cover on the aft fuselage. It also tends to occur on engine nacelles on multi-engine airplanes. Adding suitably placed VGs can reattach separated flow on a bluff after-body without any changes in the overall contour of the body.
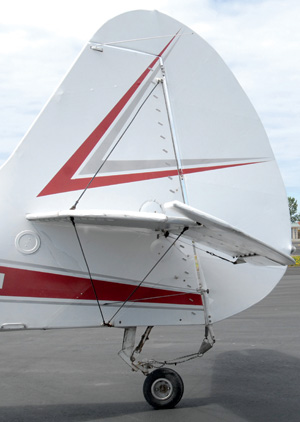
Control-Power Improvement
Sometimes, it is desirable to increase the control power of an airplane. This is particularly true of rudder power on multi-engine airplanes. If, for example, an airplane is retrofitted with more powerful engines than those for which it was originally designed, the extra power may render the rudder incapable of keeping the airplane straight in the event of engine failure. The lack of rudder power causes minimum control speed (VMC) to rise. To bring it down, more rudder power is needed.
The effectiveness of a control surface is limited by how far the surface can be deflected before the flow separates at the hinge line. If an airplane proves to have insufficient control power, the designer is faced with a problem. Increasing the maximum deflection of the controls helps up to the point where the flow over the control surfaces begins to separate. If still more control power is needed, other solutions are necessary. One is to increase the size of the control surface. This is sometimes possible, but it usually involves too much redesign to be viable.
A row of VGs just upstream of the rudder hinge line increases the amount the rudder can be deflected before separation begins. Vortex generators ahead of the rudder hinge are a feature of several of the VG kits currently marketed to tame production light twins. They also can be found on the Beech Starship, the Boeing 727 and many other production airplanes.
Maximum Lift Coefficient Improvement
Stall is caused by separation of the airflow over a wing. Reducing separation and delaying the stall to a higher angle of attack will increase the maximum lift coefficient (CLMAX) and reduce stall speed. Properly placed VGs on the upper surface of the wing can achieve this, and they may be used in several ways.
The first is to use a full-span row of VGs. This will increase the maximum lift of the entire wing. Full-span VGs are also useful in situations where contamination of the airfoil by rain, bugs or dirt can cause premature separation. This contamination problem, which is primarily a characteristic of airfoils designed to have both high lift and laminar flow, is the cause of the so-called wet canard problem that appeared on several canard and tandem-wing homebuilts some years ago.
When a high-lift laminar-flow airfoil, flying at its designed cruising angle of attack, is clean, the airflow stays attached to the upper surface all the way to the trailing edge. When rain, bugs or other contamination disturb the laminar boundary layer, there is less energy in the boundary layer as it flows over the aft portion of the airfoil, and the flow separates prematurely. If there is a flap on the airfoil, its ability to generate extra lift is severely decreased by the separation upstream of the hinge line.
If this situation occurs on a conventionally configured airplane, it is merely annoying. The stall speed of the airplane increases, and the pilot must be aware that the airplane cant safely fly as slowly as it can when clean.
If, on the other hand, the airfoil in question is the foreplane of a canard or tandem-wing airplane, the situation can be more serious. A loss of lift on the foreplane will cause a nose-down trim change. Depending on the extent of the separation caused by rain, this problem can range from merely annoying to dangerous. If the separation on the canard is severe enough, the elevator may be ineffective, and the pilot will not be able to pull the nose up-a situation that is unacceptable. The pilot must be able to prevent dives into the ground.
The solution that evolved was to place a row of VGs on the canard at about 45% chord (which was the position of the VGs on Voyager). This aerodynamic fix does not come entirely free. The VGs are in the airstream all the time, and they do produce some drag. On Voyager, the optimized VGs increased the drag of the canard by about 10%.
On a conventional airplane, using VGs over the full span of the wing can reduce the stall speed of the airplane by increasing the CLMAX of the wing. By using them only on the outer portion of the wing, the stall of the tips can be delayed, giving the airplane a gentle, root-first stall.
VG Geometry
There are two common ways of installing vane-type VGs. All of them should be mounted so that they have an angle of attack relative to the local airflow of between 15 and 20, but they may be mounted so that they are all parallel to each other, or in pairs with equal and opposite angles of attack.
The first approach, with all of the VGs parallel, is called a co-rotating installation, because all of the vortex generators shed vortices that are rotating in the same sense. The paired equal-and-opposite-angle installation is called a counter-rotating installation, because each pair of VGs sheds a pair of vortices that rotate in opposite directions.
The Voyager (and most other canard airplanes) uses a row of counter-rotating pairs of generators. The VGs on the fin of the Boeing 727 and on the canard of the Starship are co-rotating. Counter-rotating VG installations are more widely used, and they are somewhat better understood than the co-rotating installations.
One place where co-rotating VGs are preferable is on a swept surface where one half of a counter-rotating pair of VGs is likely to be at an excessively large angle of attack. For a swept surface, toed-out, co-rotating VGs are preferred.
VG Size
To effectively mix outer-flow air into the boundary layer, VGs must stick out above the edge of the boundary layer. To minimize drag, they should stick out of the boundary layer only a little. A recommended height for a first try is about 1.2 times the local boundary-layer thickness. Boundary layer thickness is difficult to determine, but it is usually on the order of 1% to 2% of the distance from the leading edge.
VG Shape and Placement
Typical vortex generators are either triangular or trapezoidal, with swept leading edges and vertical trailing edges. For co-rotating VGs, the root chord of the VG should be on the order of four times the VG height, and the distance between them in a row should be five to six times the VG height. For counter-rotating VGs, the root chord of the VG should be about 2.5 times the VG height. The distance between the centers of the two VGs in a counter-rotating pair should be about the same as the root chord of one VG. The counter-rotating pairs should be spaced about 10 VG heights apart.
Making a vortex-generator installation work effectively will require some experimentation with the size, number and spacing. If an initial installation provides the desired effect, a builder should try reducing the size and/or the number of VGs to help reduce drag. Conversely, if more effectiveness is needed, the size and/or number of vortex generators should be increased.