As I write this article, I am involved in the flight test of a new airplane that has fallen prey to a common problem in developmental aviation. The airplane was designed, at the request of the customer, around a relatively new and unproven engine. By the time the prototype was ready, the engine had failed, and its manufacturer had gone out of business.
We are now faced with the problem of adapting a different, less suitable but available, engine to the airframe and making the airplane work satisfactorily with the new powerplant. At the same time, I am watching the tribulations of a friend dealing with a converted automotive engine on his project. These experiences serve to remind me that one of the most critical decisions a designer makes is the choice of engine to power a new airplane.
Wainfan’s Second Law of Applied Aerodynamics states: "It’s always propulsion’s fault." While this is a bit of an exaggeration, it does highlight how important the propulsion system is to the success of the airplane as a whole. The history of aviation is littered with interesting, and fundamentally sound, airframes that were crippled by engine problems.

The Lockheed Constitution.
There were two major waves of problems, one during the latter half of WW-II, and a second in the 1950s. The WW-II problems happened when several high-powered piston engines being developed to replace the generation of engines that powered the P-51 and its contemporaries failed to develop the promised power or reliability. Many interesting and innovative airplanes, including the Curtiss XP-55 Ascender, Northrop XP-56 Black Bullet, Vultee XP-54 Swoose, Lockheed XP-59 Chain Lightning and the much larger Lockheed Constitution transport were designed around these promised engines. When the engine development ran into problems, the airplanes were either flown with standard lower-power engines as substitutes, or with de-rated prototypes of the new engines. In all of these cases the new airplanes suffered because they were underpowered, and none of them proved successful.

The Northrop XP-56 Black Bullet.
The second major round of such problems happened during the early part of the jet era when several engines, notably those manufactured by Allison and Westinghouse, failed to deliver the promised thrust. Again, several promising airplane designs died or had short, unsatisfactory service lives because their engines did not deliver the thrust and reliability needed. The most extreme case was a Navy fighter built by McDonnell Aircraft that was so underpowered it could not take off from Lambert Field in St. Louis (the home of McDonnell Aircraft) in the summer. The first batch of these airplanes was shipped new from the plant by barge and then trucked to various bases to be used as non-flying maintenance trainers.
All of these sad stories result from what is now recognized as one of the most common traps in airplane design: creating an airplane that is dependent on an unproven engine to succeed. The engine is in many ways the heart of the airplane, and both performance and safety depend on it.
Performance
When the designer lays out an airplane, the design is predicated on the engine being able to produce a certain amount of power (or thrust), weighing a given amount and consuming a given amount of fuel per hour. If the engine falls short in any of these areas, it hurts the performance of the airplane.
Power
The power of the engine affects speed, climb and ceiling. The less power, the slower the airplane will fly. Although speed seems to be the aspect we worry about the most, loss of cruise speed is probably the least important consequence of a power shortfall, particularly for light airplanes. To the first order, the top (or cruise) speed of a general aviation airplane is proportional to the cube root of the power of the engine. What this means is that it takes a large change in power to get a little increase in speed. For example, to increase speed 10% we must increase power by 33%. Conversely, if the engine falls 10% short of its expected power output, cruise speed will fall by only about 3.5%. While this is notdesirable, it is also not catastrophic unless you are racing.
Climb is a very different story. The rate of climb of an airplane is a function of specific excess power. Specific excess power is the excess power per pound of airplane, or the excess power divided by the gross weight. Excess power is the power available from the engine over and above the power required to maintain level flight. For example, if an airplane with 100 horsepower is flying at an airspeed that takes 75 hp to overcome drag, then it has 25 excess horsepower. The actual excess thrust horsepower available is less than 25 because the propeller is not 100% efficient.
The important point here is that the excess power is considerably less than the full power of the engine because of the power required to overcome drag and maintain airspeed. Only the excess power above that is available to make the airplane climb. Accordingly, a power shortfall will affect rate of climb much more than it affects speed. The percentage loss in climb performance will be much greater than the percentage shortfall in rated engine power.
A shortfall in takeoff and climb performance has dire effects on the airplane’s safety and utility. If the engine won’t produce enough power, then the only way to regain the climb performance needed to operate out of the specified runways at the required density altitude is to reduce weight. Unfortunately, once the airplane is built, the only way to do this is to offload payload or fuel. This means the only choice left is to carry less or not fly as far, neither of which is desirable.
Weight
Weight is the enemy of performance. Every pound of airplane empty weight represents a pound of fuel or payload that the airplane can’t carry. An overweight engine presents the designer with the decision of letting the gross weight rise or reducing the fuel or payload the airplane carries. Increasing gross weight reduces rate of climb, hurts takeoff performance and may require structural beef-up that will increase weight even more. Once the airframe has been built, there is little choice but to reduce useful load to stay within the gross weight limit of the airframe if the engine is overweight.
An overweight engine can also cause center of gravity problems. The weight and balance of the airplane is calculated during design based on the predicted weight of the parts that will go into it. If the engine is overweight, the empty CG of the airplane will be closer to the engine than the designer intended. On a tractor, this will make it nose heavy, and on a pusher, increasing engine weight will make it tail heavy. Compensating for this CG shift can be a problem. On some airplanes, it is possible to build a new, shorter engine mount that moves the engine closer to the desired empty CG. This might require a new cowling and make access to the engine accessories difficult, but it is the best solution available if it can be accomplished.
If changing the engine mount does not solve the CG problem, the only other solution available is to add ballast to bring the CG back into the acceptable range. While this might solve the CG problem, the poor airplane takes a double weight hit: the extra engine weight and the weight of the compensatory ballast. As we have already seen, this added weight does bad things to the utility of the airplane.
Fuel Consumption
One of the most important characteristics of an engine is the amount of fuel it burns. The designer uses the predicted fuel consumption of the engine to size the fuel tanks and determine the total weight of fuel the airplane must carry to get the desired range. If the engine burns more fuel than advertised, we are faced with the familiar two choices: accept decreased performance (in this case, less range), or make the airplane heavier by adding more fuel capacity.
Optimistic fuel-consumption numbers are a chronic problem in the Experimental aircraft world, particularly for uncertified engines. I have had the experience of dealing with an engine that had fully twice the advertised fuel burn per hour at cruise power. Even using a certified, well-documented engine, a designer needs to be careful. The published numbers are for a new, in-tune engine being flown with an optimally set mixture. In the real world fuel consumption is probably a bit higher. It is a good idea for a designer to do some investigation about how an engine under consideration performs in the field before defining fuel capacity. This is difficult to do with a new or Experimental engine, but it’s relatively easy to do for certified engines by talking to pilots who fly that type of engine regularly, or by doing some experiments with an airplane powered by the engine.
Reliability
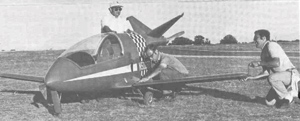
When the engine the BD-5 was designed around proved unreliable, a solution couldn’t be found before the company went bankrupt.
An unreliable engine leads to a dangerous airplane. No matter what other virtues an engine might have, a power loss in flight is always a bad thing. The engine must be reliable enough that the pilot can trust his life, and the life of his passengers, with it. In the Experimental aviation world it is tempting to sacrifice some engine reliability to try to gain another advantage. In the quest for reduced cost or increased performance, we sometimes turn to new, unproven powerplants. Some of these turn out to be workable, but some do not. Remember that flying with a relatively untried engine is inherently risky, and proceed with great caution.
Avoiding the Trap
A designer has two strategies available to avoid the "bad engine" trap. The simplest is to design the airplane around a commonly available, well-proven engine. This is the conservative approach, but the advantage is that it usually works. If there is a strong reason to use a newer, less proven powerplant, it is important to leave yourself an out. If the airplane configuration is so tightly integrated around the new engine that it is the only one that will fit or balance, then the success of the airplane is completely dependent on the success of the engine.
A classic example of this in the kit airplane world is the BD-5, which was designed to use a lightweight two-stroke engine buried in the fuselage, driving a pusher propeller. The airplane was small and light, so it was not tolerant of increases in engine size or weight. The engine the airplane was originally designed around proved to be unreliable, and several attempts to substitute other engines followed. None of these proved particularly successful, and Bede Aircraft went bankrupt before the engine problems could be sorted out, leaving a few flying airplanes and a large number of partially built kits behind. Today, a few BD-5s are flying, powered by whatever engines their determined owners were able to make fit.
The lesson of the BD-5 and similar sad stories is to make sure that your design has enough reserve volume and weight capacity to install a substitute, more well-established engine if your "new and better" engine proves not to be better, or even acceptable.
This versatility is one of the major arguments in favor of podded engines on jets. It is relatively easy to change engine types on a jet by building a new engine pod and pylon. An example of this feature in action is the Eclipse light business jet. The Eclipse was originally designed around a Williams engine being developed at the same time as the airplane. The prototype flew with the Williams engines, but the combination of those engines and that airframe did not work well, and the airplane was clearly underpowered. The Eclipse project was saved (at least temporarily) by the ability to substitute different, more powerful engines, housed in new engine pods, without having to make major changes to the airframe. Even though the delay caused by the engine problem was a major contributor to the company’s eventual bankruptcy, the ability to change engines relatively easily made it possible for the Eclipse 500 jet to be a technically acceptable aircraft.
Designing an airplane around a "paper" engine is a common path to failure. Make sure your design has a Plan B powerplant that you can buy today.
Barnaby Wainfan is a principal aerodynamics engineer for Northrop Grummans Advanced Design organization. A private pilot with single engine and glider ratings, Barnaby has been involved in the design of unconventional airplanes including canards, joined wings, flying wings and some too strange to fall into any known category.